Laboratory of Membrane Biology and Biophysics
Research in my lab centers on ATP-binding cassette (ABC) transporters, a diverse group of membrane proteins integral to almost every biological process. In prokaryotes, these proteins are critical for survival. In humans, ABC transporters make up one of the largest gene families, and more than a dozen genetic diseases have been traced to ABC transporter defects. ABC transporters are also central to multidrug resistance in many pathogenic bacteria and in tumor cells. By pursuing structural and mechanistic studies of ABC transporters, we hope to understand how nature utilizes the chemical energy of ATP hydrolysis to perform work—transporting substrates against their chemical gradients.
We are currently focusing on the following questions:
How anti-cancer drugs are ejected by ABC transporters
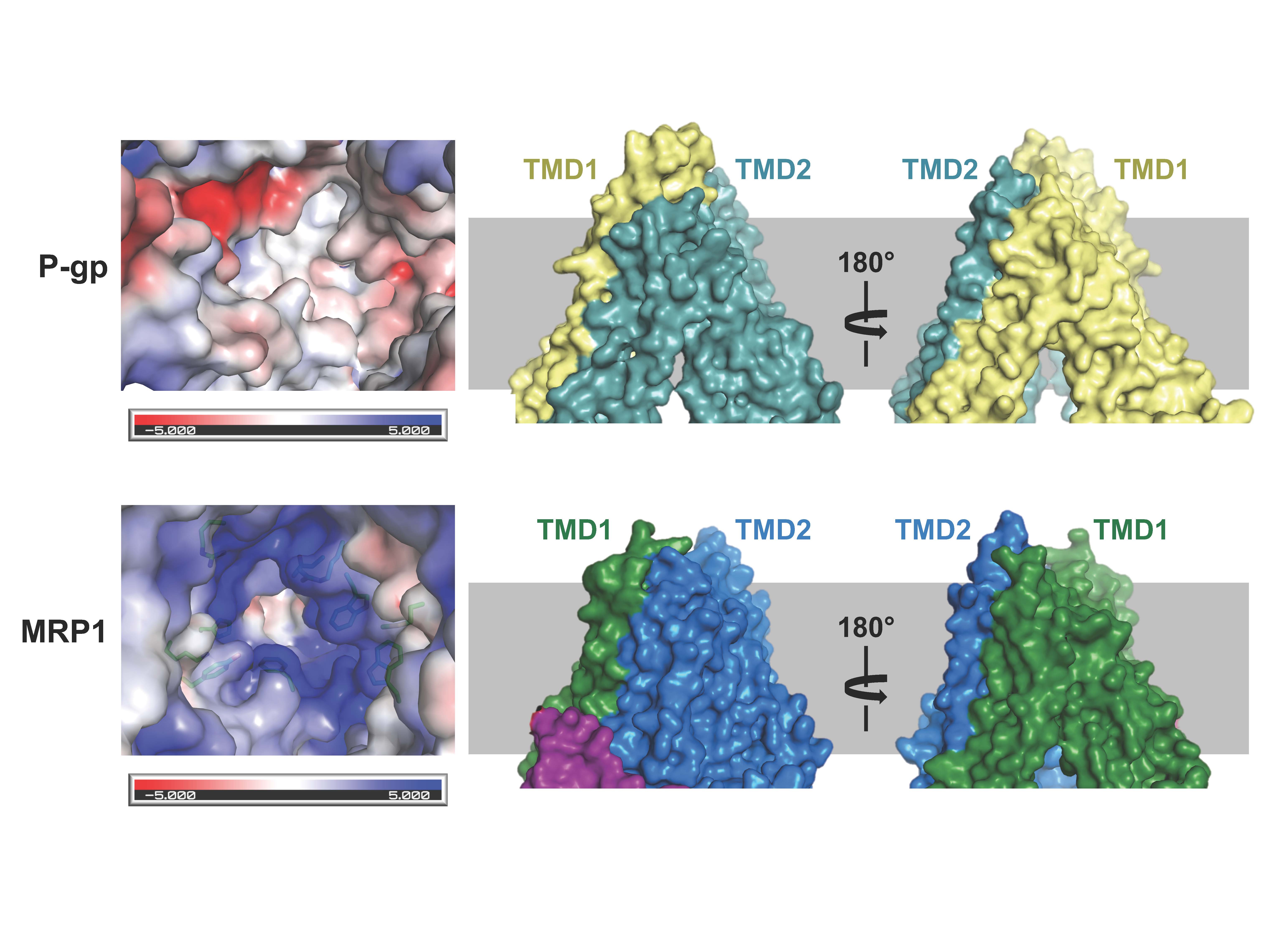
Figure 1. Structural comparison of the two multidrug pumps (see Johnson and Chen, Cell; 2017)
Click here for a movie showing substrate recognition by MRP1.
Cancer cells develop resistance to a large number of chemically diverse compounds, a phenomenon known as multidrug resistance (MDR). In 1976, Juliano and Ling identified a glycoprotein enriched in colchicine-resistant cells but not in wild-type cells. The protein was named “P-glycoprotein” (P stands for permeability) because it was thought to confer drug resistance by making the membrane less permeable. This was a reasonable conclusion at the time, as it is difficult to imagine how a single transporter could recognize many unrelated drugs. It is now known that P-glycoprotein is an ABC transporter whose physiological function is to protect sensitive tissues and the fetus from toxicity.
How antigens are transported and loaded onto MHC-I molecules
Our adaptive immunity depends on antigen presentation to cytotoxic T-cells by means of major histocompatibility complex (MHC) class I molecules. Endogenous antigens, degraded by the proteasome, are transported into the ER lumen by the ABC transporter TAP.
Subsequently, the peptides are loaded onto nascent MHC class I molecules by a macromolecular peptide-loading complex comprising TAP, MHC class I, and several auxiliary factors. Peptide-bound MHC class I molecules are exported to the cell surface for antigen presentation. Defects in TAP account for immunodeficiency and tumor development. To escape immune surveillance, some viruses have evolved strategies to either down-regulate TAP expression or directly inhibit TAP activity. We are pursuing structural studies of the TAP transporter and exploring how viral factors modulate TAP function. A long-term, ultimate goal for us is to build the large assembly of the peptide-loading complex.
Figure 2. The MHC class I antigen presentation pathway.
Click here to see the structure of TAP inhibited by a viral protein.
The root cause of cystic fibrosis
Cystic fibrosis is the most common lethal genetic disorder in populations of Northern European descent, affecting one out of every 2500 newborns. It is caused by mutations in a single gene, the cystic fibrosis transmembrane conductance regulator (CFTR).
CFTR belongs to the ATP-binding cassette (ABC) superfamily, but it is unique among ABC proteins in that it functions as an ion channel. Whereas other ABC transporters utilize the chemical energy of ATP hydrolysis to transport substrates against their chemical gradients, CFTR conducts anions down their electrochemical gradient.
By combining structural biology, electrophysiology, and medicinal chemistry, we hope to contribute to treating—and eradicating—this devastating disease.
Figure 3. Structure of human CFTR.
The E. coli maltose transporter, a prototype for ABC transporters
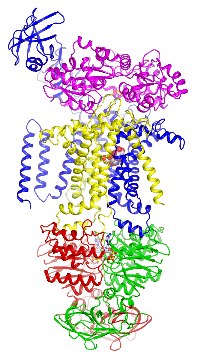
The maltose transporter is a nutrient importer that has been extensively studied for over 40 years as a model system for membrane transporters. My lab has previously determined the crystal structures of the maltose transporter in different stages of the transport cycle, including the inward-facing resting state; the substrate-induced pre-translocation state; the outward-facing catalytic intermediate; and the EIIAGlc-inhibited state.
Based on these structures and the rich genetic and biochemical data in the literature, we are now able to describe the atomic details of how ATP hydrolysis enables maltose transport; the chemistry of ATP hydrolysis; how different substrates are recognized; and, finally, how bacteria regulate maltose uptake to optimize growth. The mechanistic insights gleaned from the maltose transporter can also be applied to many other ABC transporters.
The maltose transporter is a nutrient importer that has been extensively studied for over 40 years as a model system for membrane transporters. My lab has previously determined the crystal structures of the maltose transporter in different stages of the transport cycle, including the inward-facing resting state; the substrate-induced pre-translocation state; the outward-facing catalytic intermediate; and the EIIAGlc-inhibited state.
Based on these structures and the rich genetic and biochemical data in the literature, we are now able to describe the atomic details of how ATP hydrolysis enables maltose transport; the chemistry of ATP hydrolysis; how different substrates are recognized; and, finally, how bacteria regulate maltose uptake to optimize growth. The mechanistic insights gleaned from the maltose transporter can also be applied to many other ABC transporters.