Robin Chemers Neustein Laboratory of Mammalian Cell Biology and Development
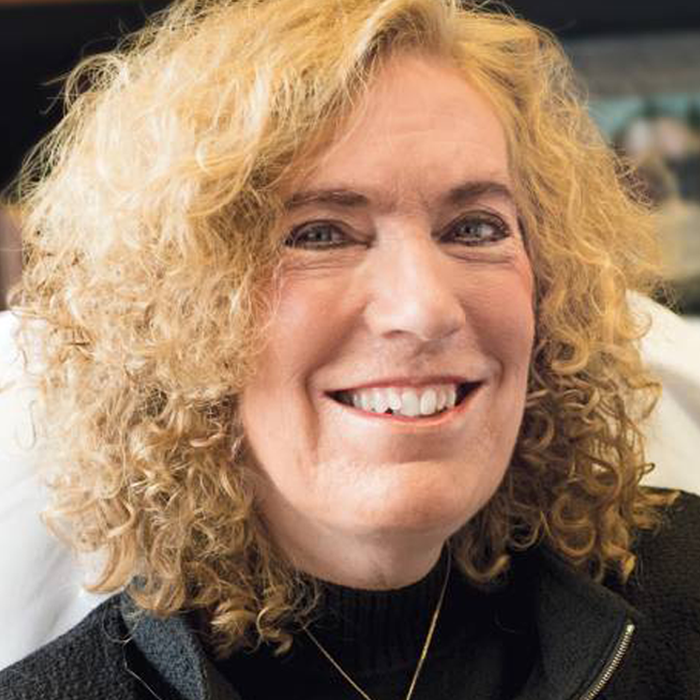
Investigator, Howard Hughes Medical Institute
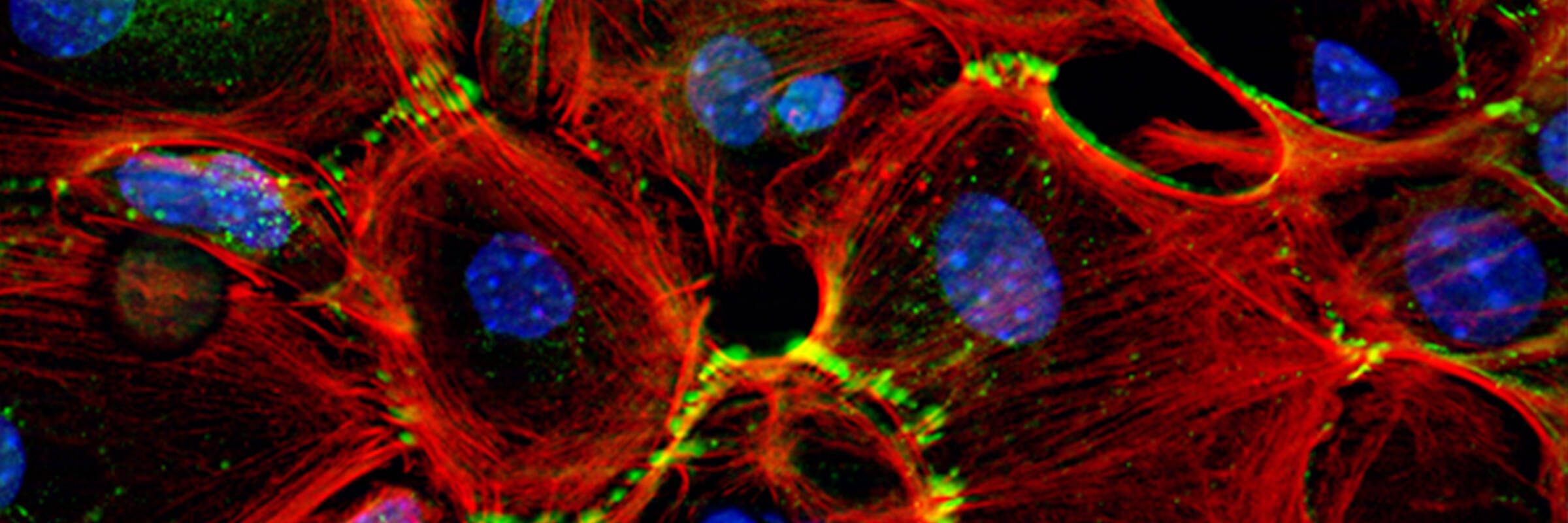
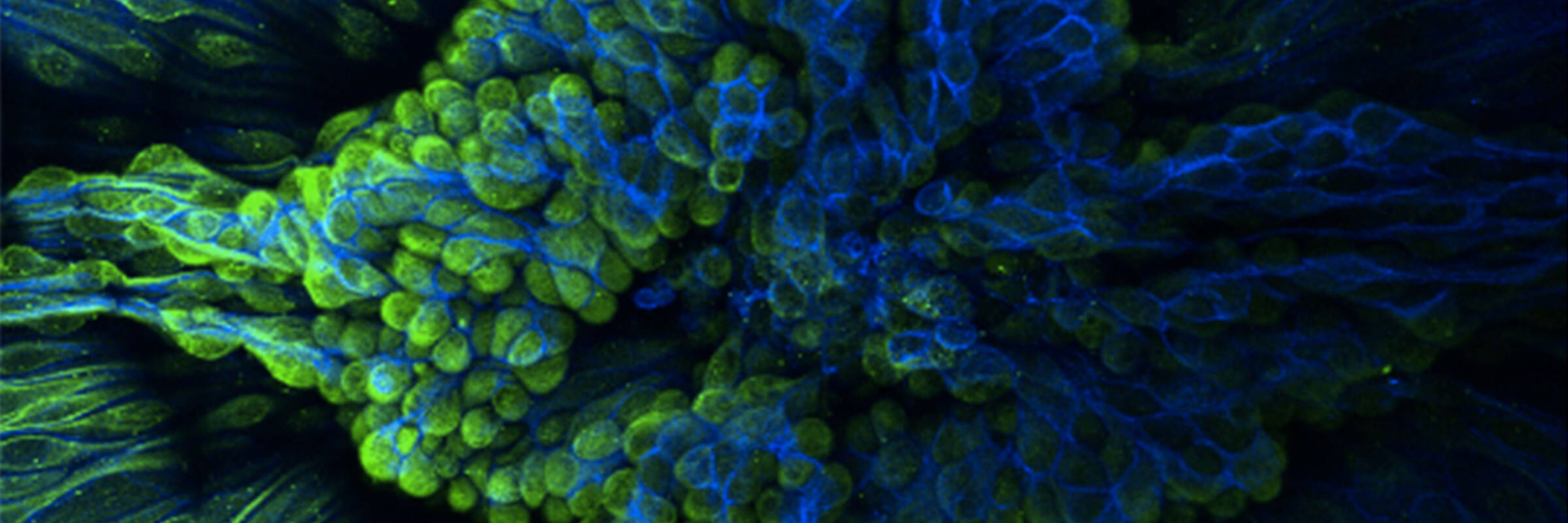
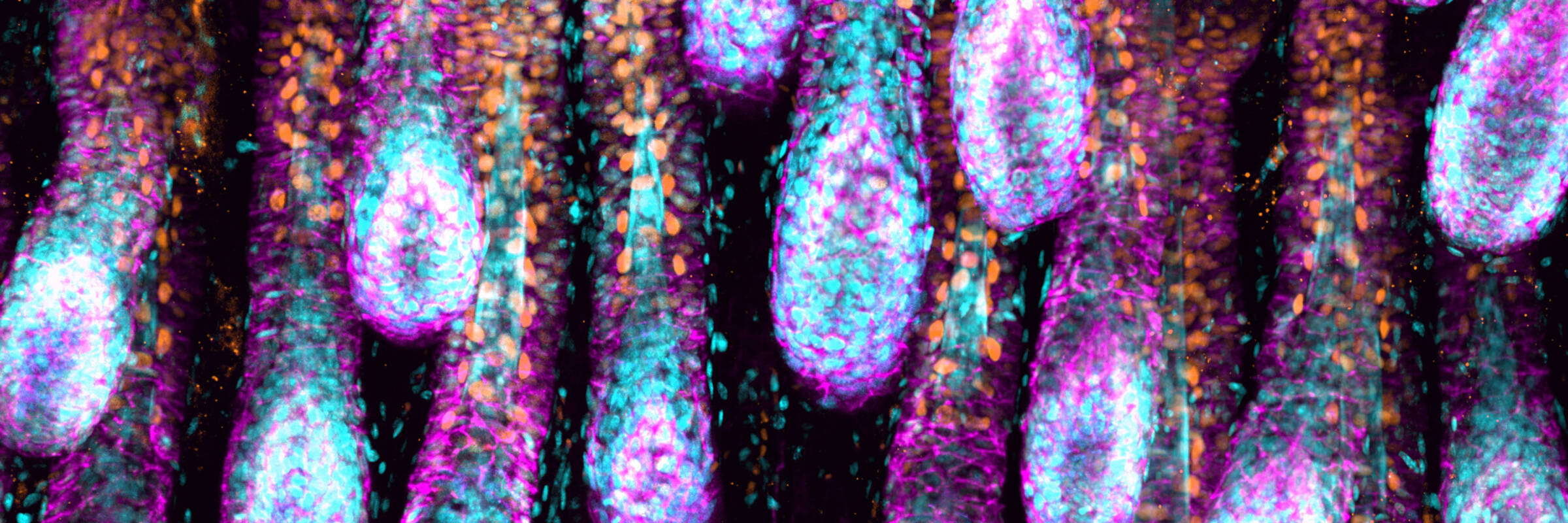
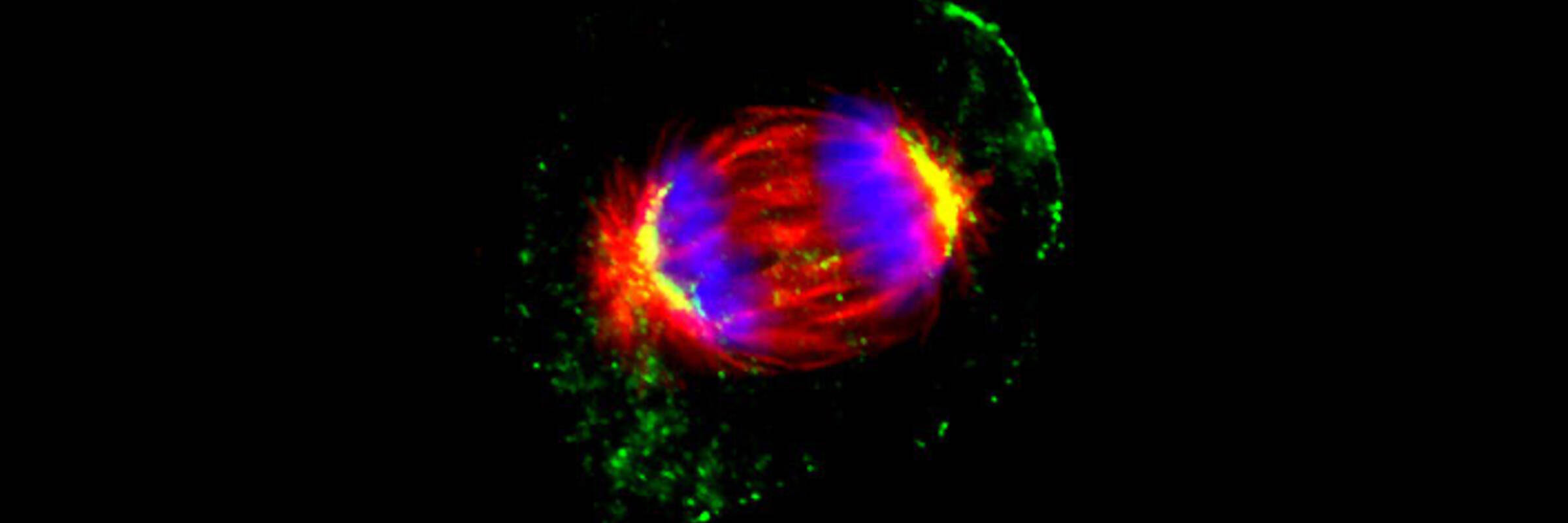
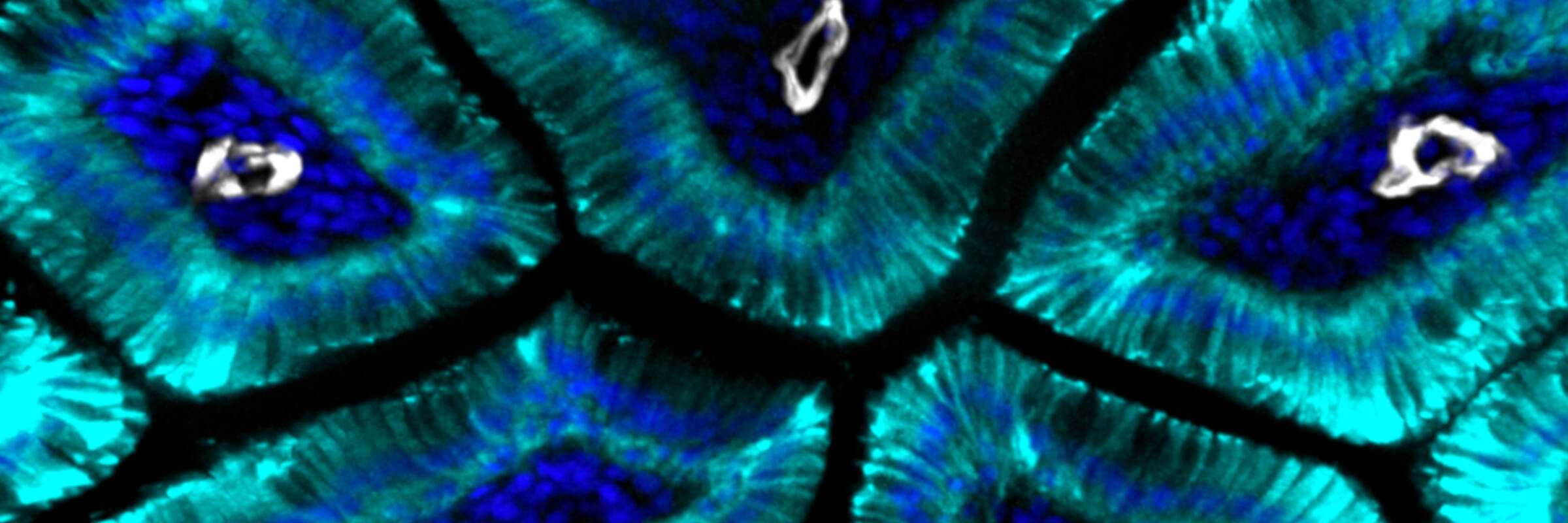
The skin epidermis is what allows us to survive as terrestrial beings. It acts as a saran wrap seal to our body surface, excluding microbes and retaining body fluids. Subjected constantly to mechanical stress, epidermal cells protect themselves by producing an elaborate cytoskeleton that connects to specialized cellular junctions and enables the cells to form adhesive sheets of resilient tissue. The epidermis also produces protective appendages, such as feathers in birds, scales in fishes and hair follicles in mammals. Human skin has evolved to have sweat glands, enabling us to regulate body temperature and survive over broader climates than other mammals. To withstand normal wear and tear, epidermis constantly self-renews, making it one of the body’s main reservoirs of stem cells. When the skin’s barrier is breached in injury, epidermal stem cells must also communicate with resident immune cells to cope with inflammatory immune cells, prevent infection and repair the damaged tissue. Finally, at the body’s surface, skin stem cells are also subjected to harmful UV rays, and not surprisingly, epidermal cancers are the most common of all human cancers.
In the Fuchs’ laboratory, we are trying to understand how tissue stem cells of mammalian skin give rise to the epidermis, hair follicles and sweat glands. We study how skin stem cells respond to different signals from their environment and orchestrate the changes in chromatin, transcription, translation, cell polarity, adhesion and cytoskeletal dynamics in normal skin development, homeostasis and wound repair. Elucidating the normal process of tissue dynamics is providing us a foundation to understand how these processes go awry in aging as well as in genetic skin diseases, including chronic wound-healing, chronic inflammatory disorders and cancers. We employ novel mouse genetics to perform genetic screens and test the functional relevance of our findings. However, we are also aided in our ability to culture human and mouse skin epithelial stem cells under conditions where they retain their ability to regenerate tissue upon engraftment. In our research approaches, we utilize a broad range of high throughput techniques as well as cell, molecular and developmental biology. The unprecedented speed and ease of using skin as a model system has allowed us to gain fundamental insights into tissue stem cells in health and disease.